When designing structural components, a variety of environmental factors need to be taken into account depending on the geographical location.
Ice accumulation on structures is rarely a dominant factor, but it can be crucial in structural design. Although often overlooked, it is essential to consider icing to ensure full compliance with building code requirements.
By understanding the critical aspects of icing, engineers and architects can create safer and more resilient buildings capable of withstanding harsh winter conditions.
In this article, we will explore the concern of structural icing, explain why it is important, and delve into what building codes say about it. We will discuss the potential risks associated with ice accumulation on structures and provide practical guidance on how to address these challenges in the design process.
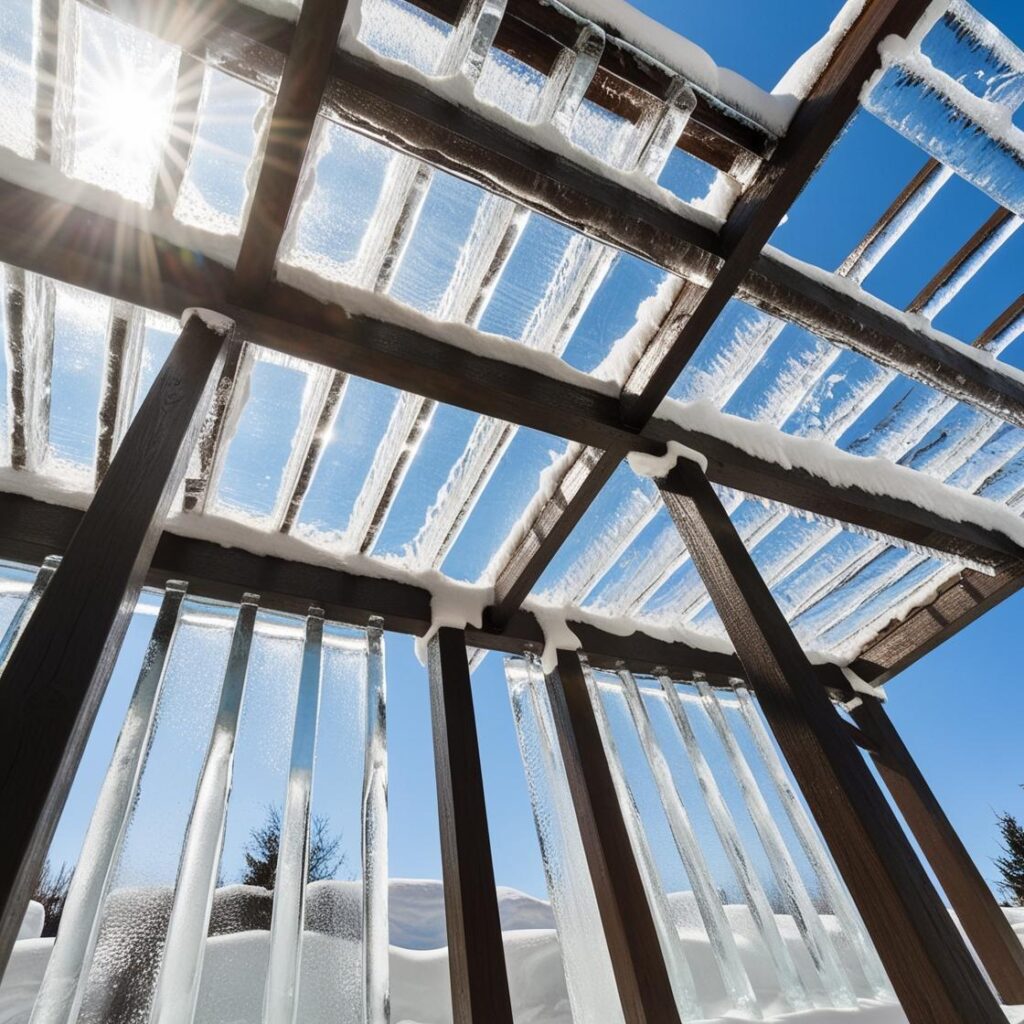
Why is It Crucial to Consider Icing in Design?
What is Icing?
Icing refers to the accumulation of ice on structures, especially in regions where temperatures fluctuate above and below freezing. While icing can occur in colder areas, it is often most problematic in places where temperatures swing, allowing rain to fall as water before freezing. This process can lead to additional rain falling onto already frozen surfaces, preventing the ice from thawing. Icing occurs when moisture freezes on surfaces during cold weather, often due to freezing rain, snow, high humidity, or condensation. For buildings, icing can accumulate on roofs, beams, and other components, adding extra weight that can strain the structure. This ice accumulation is crucial to consider during the design and construction process, as it can impact a building’s safety and durability. By understanding icing, we can ensure that structures are equipped to handle the challenges posed by winter weather.
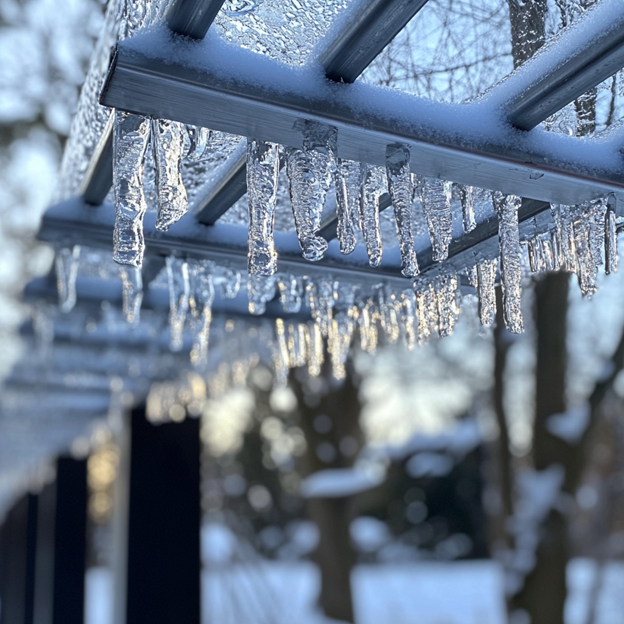
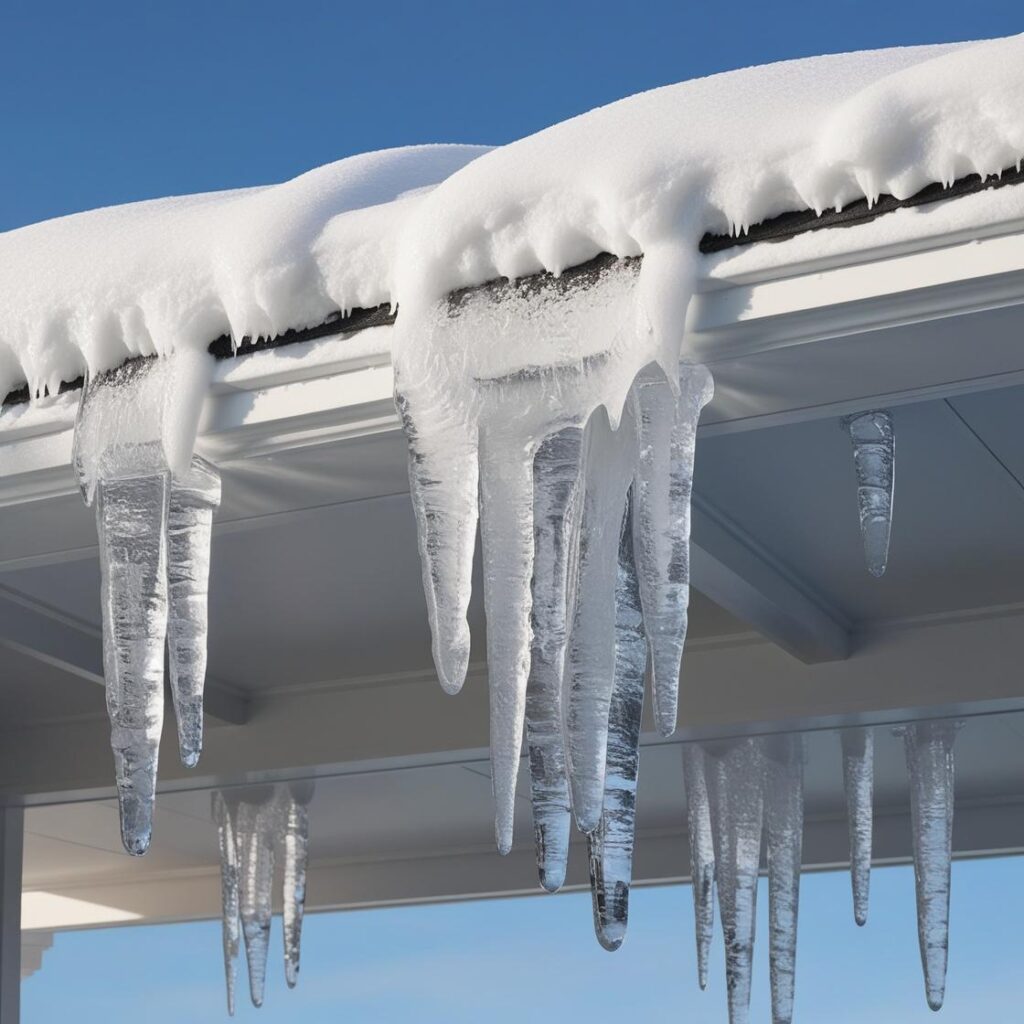
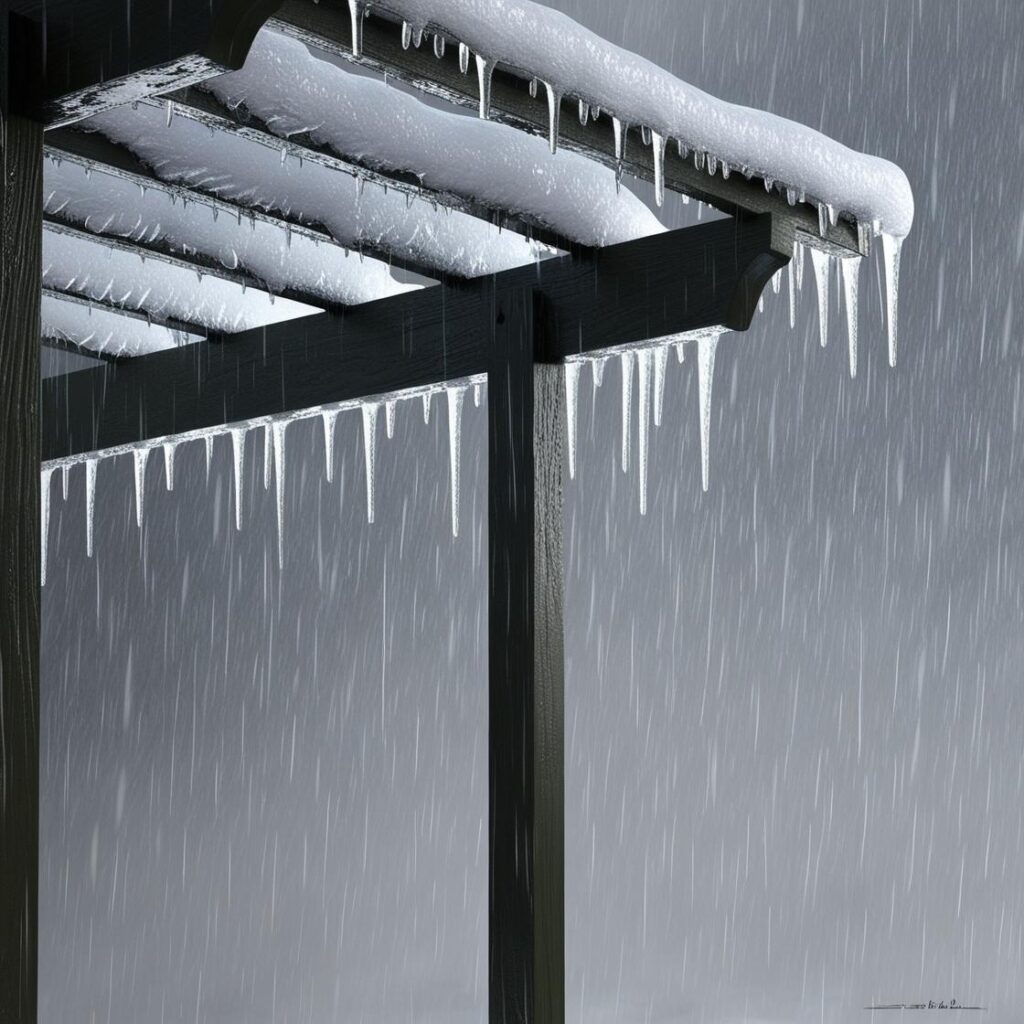
Key Risks and Concerns
Icing poses several risks to structures, particularly in regions that experience severe winter weather. The accumulation of ice adds significant weight to roofs, bridges, and other structural components, potentially exceeding their load-bearing capacity and leading to structural failures. This not only results in costly repairs but also creates safety hazards for occupants and passersby, as falling ice can cause injuries. Additionally, icy conditions can lead to slippery surfaces, increasing the risk of accidents. Ice buildup can disrupt utility services, straining power lines and causing outages.
Case Study - The Texas Icing Power Crisis
One clear example of these risks happened during the Texas Power Crisis in February 2021, when icing from a severe winter storm exposed the weaknesses in the state’s energy infrastructure.
In February 2021, Texas faced a severe winter storm that led to a catastrophic power crisis, exposing vulnerabilities in the state’s energy infrastructure. One significant factor contributing to this crisis was icing, which caused widespread outages and highlighted the urgent need for improvements in weather preparedness.
The storm brought record-low temperatures, snow, and ice, leading to the accumulation of ice on power lines, transformers, and other electrical infrastructure. The weight of the ice caused power lines to sag and break, resulting in widespread power outages that affected millions of residents. Additionally, many power plants, particularly those relying on natural gas, were unable to operate efficiently due to freezing temperatures and the icing of equipment.
Many power generators were not adequately prepared for extreme winter conditions, and the lack of insulation and heating mechanisms for critical equipment left them vulnerable to icing. As a result, a significant number of power generation facilities were forced to shut down, leading to a dramatic reduction in available electricity during a time of peak demand.
As we move forward, it’s important to take icing and its effects seriously in our planning and design efforts. By considering these factors, we can create safer and more resilient structures that better protect our communities during unexpected weather events.
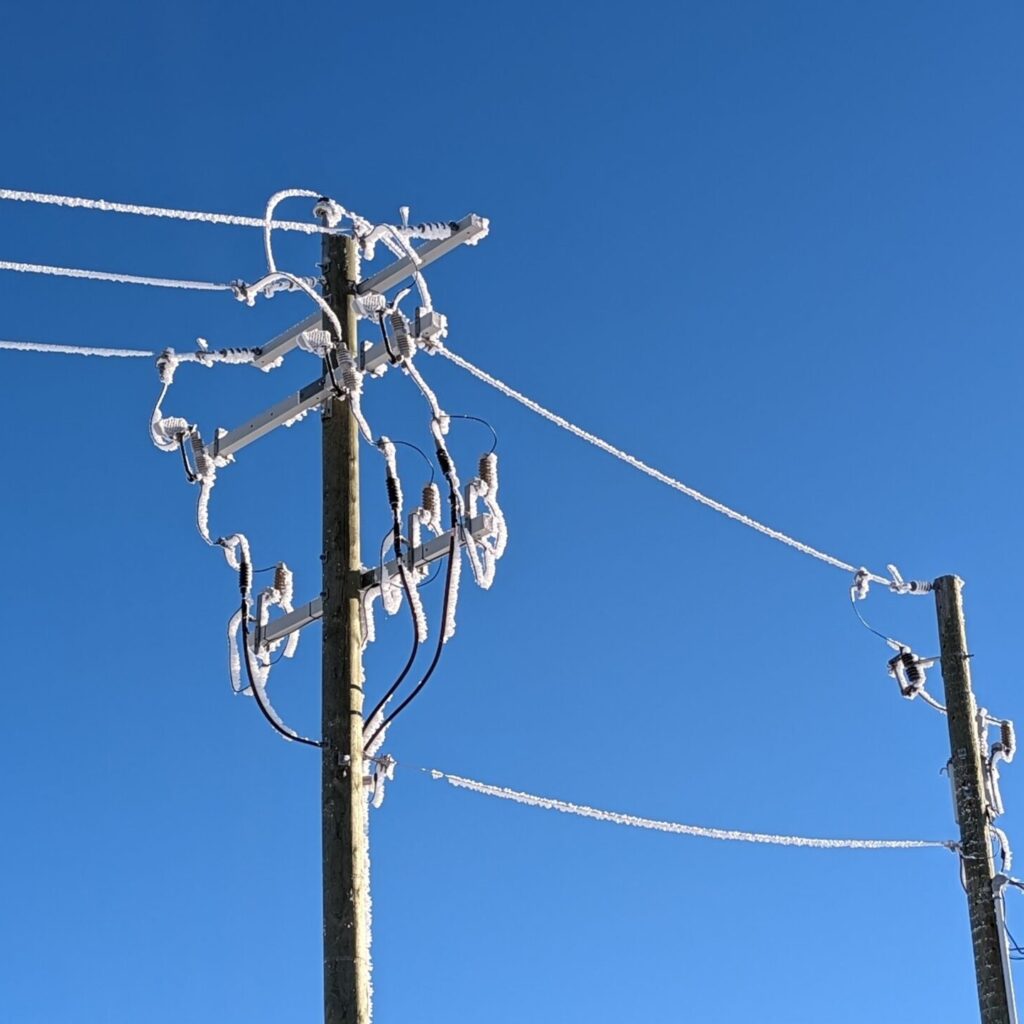
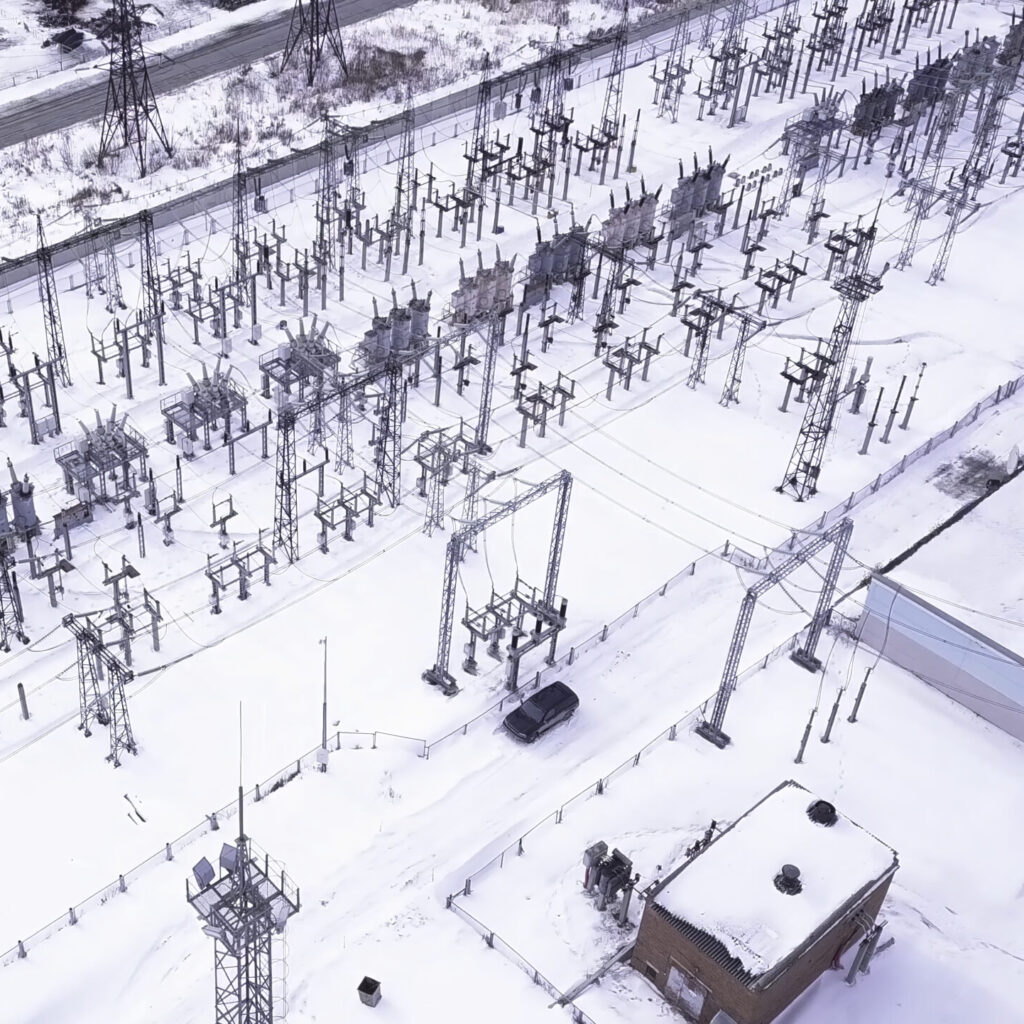
Icing in Building Codes: What You Need to Know
A Closer Look at Icing Regulations in ASCE 7
Building codes play a vital role in the design and construction of safe and resilient structures, particularly in areas prone to extreme weather conditions such as icing. Adhering to these regulations ensures that buildings are designed to meet specific safety standards, especially when determining loads. The American Society of Civil Engineers (ASCE) emphasizes the significance of icing in Chapter 10 of its guidelines, highlighting the need for engineers to account for potential ice accumulation when calculating load-bearing capacities. This consideration is crucial for ensuring that roofs, beams, and other structural elements can withstand the added weight of ice without compromising safety. It is important to note that these provisions focus specifically on structural applications rather than mechanical systems; while components like levers and motors may experience freezing, they do not typically lead to structural failure.
The icing regulations outlined in ASCE 7 specifically refer to ice-sensitive structures. These structures are defined as those for which atmospheric icing loads significantly influence the design. This category includes various structures, such as communication towers, overhead lines, bridges, and cranes, all of which are particularly vulnerable to ice accumulation. It may be up to an engineer to determine if a structure is ice-sensitive and should be designed for structural icing loads. Given this vulnerability, it is crucial to design these structures with careful consideration of ice loads. By understanding the unique requirements of ice-sensitive structures, engineers can ensure their safety, reliability, and functionality during harsh weather conditions.
How to Calculate Ice Loads ?
Here is a brief step-by-step guide to calculating ice loads. This summary is based on the ASCE 7-22 Chapter 10 guidelines. For more detailed information and guidance on handling various loads, you can check out our design calculators, contact us for site-specific projects, or reach out to us with your inquiries. Click here for more info.
The main idea when calculating ice loads is to determine the additional weight that ice buildup will impose on a structure. This process begins by calculating the approximate perimeter of the shape being iced, such as the cross-sectional perimeter of a beam, pole, or other structural element exposed to icing conditions. This measurement gives the boundary within which ice will accumulate.
Next, you need to refer to the ice load map provided in ASCE 7-22 Chapter 10. This map details the expected ice thickness (t) for various regions, based on historical weather data and regional climate conditions. By finding your location on the map, you can determine the average ice thickness (t) that your structure might encounter during an icing event.
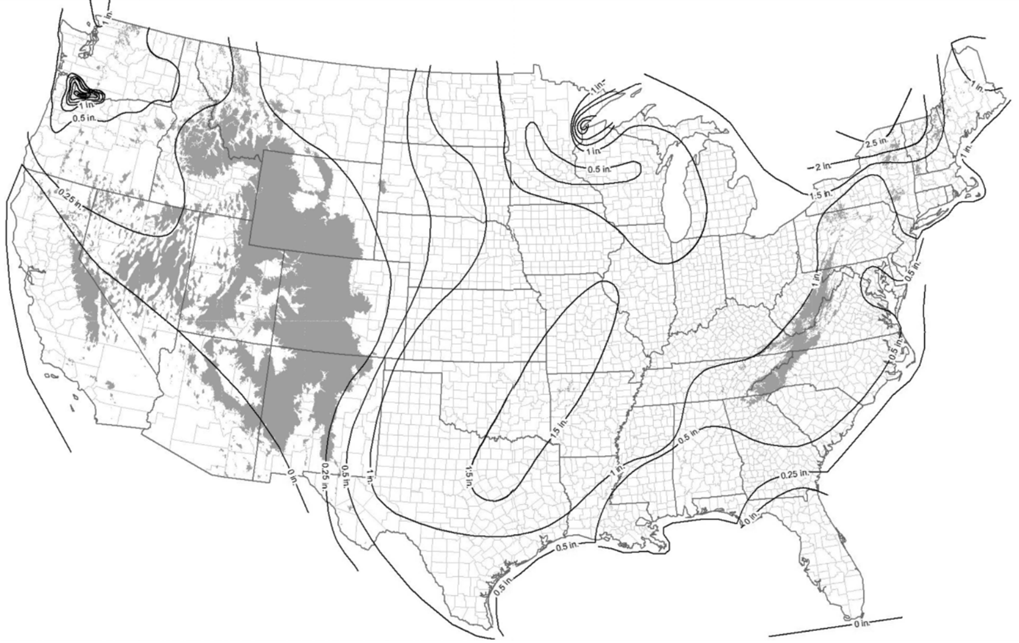
With the perimeter of the structure and the expected ice thickness, you can then calculate the total volume of ice that will build up on the structure. Two different calculation scenarios can be encountered: prismatic shapes and flat plates or surfaces. Each scenario requires a slightly different approach to determine the total volume of ice.
Ice Loads on Prismatic Shapes
Ice Loads on Flat Plates and Surfaces
For structural shapes, prismatic members, and similar elements like guy wires, the ice volume is calculated using Equation 10.4-1 from ASCE 7.
Ai = ( π .td / 12 ).( Dc + td / 12 )
The Characteristic Dimension (Dc) refers to the largest diagonal dimension of the shape. Those familiar with extrusion design will recognize this dimension as the “circle size” of a particular die.
Once the cross-sectional Area of Ice is determined using the appropriate formula, you can calculate the ice load by multiplying the area by the design ice density, which is a minimum of 56 lbs/ft³. This will give you the ice load in pounds per foot for the prismatic member.
For flat plates and large three-dimensional objects, the volume of ice is calculated using Equation 10.4-2.
Vi = ( π .td / 12 ).As
For a flat plate, As shall be the area of one side of the plate; for domes and spheres, As shall be determined by:
As = πr2
Here are two allowed reductions for calculating ice volume on flat plates:
- – For vertical plates, you can reduce the ice volume by multiplying it by 0.8.
- – For horizontal plates, you can reduce the ice volume by multiplying it by 0.6.
Once you have the ice volume, multiply it by the design ice density (at least 56 lbs/ft³) to get the total weight of the ice on the structure. If you need the weight per square foot for design purposes, simply divide the total weight by the surface area.
For more detailed information and guidance on handling various loads for your future projects, check out our online design calculators, contact us for site-specific projects, or reach out to us with your inquiries.
Optimizing Ice Design: The Benefits of Reduced Wind Load
An important consideration for engineers when designing for ice loads is the use of reduced wind loads. Designing for both maximum wind and ice at the same time can lead to unnecessary complexity and increased costs. Instead, it’s beneficial to focus on reduced wind loads during ice design. This is because ice typically accumulates when wind conditions are milder. By applying this approach, engineers can create safer and more efficient designs that meet structural requirements without overbuilding. This strategy not only enhances the performance of the structure but also helps in managing project costs effectively.
In Conclusion
Understanding and accurately calculating ice loads is essential for safe and efficient structural design.
By considering factors such as reduced wind loads, engineers can optimize their designs to better withstand the unique challenges posed by ice accumulation.
As you tackle load calculations for your future projects, remember that our online design calculators and expert consultations are here to support you.
If you have any questions or need assistance with a specific project, feel free to reach out—we’re ready to help! And don’t forget to explore our other knowledge base articles for more helpful tips and insights.
Article provided by Chris Arbelbide.
Last Update: December 17, 2024
Tell us how can we improve this post?
Related Knowledge Base Posts -
No related posts found !